1 GPa 급 고강도강 용접금속에서의 침상형 페라이트 핵생성 거동
Nucleation Behavior of Acicular Ferrite in 1 GPa Class High Strength Steel Weld Metal
Article information
Abstract
In this study, the nucleation behavior of acicular ferrite in 1 GPa class high strength steel weld metal was investigated. A 1 GPa class as-deposited weld metal specimen was prepared by single-pass welding using a prototype flux-cored wire, and a reheated weld metal specimen was obtained using Gleeble simulation technique. The typical inclusion in both specimens was composed of MnTi2O4 and amorphous. It was found that the inclusion potency for acicular ferrite nucleation was maximized in the as-deposited weld metal because of both the formation of Mn-depleted zone (MDZ) near the MnTi2O4 and the low interfacial energy between the MnTi2O4 and ferrite. In the reheated weld metal specimen, it was observed that the inclusion potency decreased possibly due to the homogenization of Mn in the MDZ that occurred during reheating thermal cycling.
1. 서 론
최근 원유와 가스 등 에너지원을 확보하기 위해 심해와 한랭지를 대상으로 한 원거리의 자원개발 수요가 증가하고 있다. 극한환경에서의 에너지원의 효율적인 채굴과 운송을 위한 선박과 해양구조물의 대형화 및 파이프라인의 대구경화 등이 요구되고 있으며, 이에 따라 다양한 산업분야에서 높은 강도와 저온인성을 갖는 구조용 강재에 대한 수요가 급증하고 있다. 최근에는 압연 및 열처리 기술을 통해 높은 저온인성을 갖는 1 GPa 급 고강도 강재의 개발이 이루어지고 있다1,2). 파이프라인 분야에서 최근 개발된 API-X120 강재의 경우 931 MPa 이상의 인장강도 요건을 가지며 실제로는 대부분 1 GPa를 상회한다3). 한편 용접부의 경우 열처리 기술 적용이 현실적으로 어렵기 때문에 고강도 및 고인성을 확보하기 위한 용접재료 합금설계 기술에 관한 연구가 꾸준히 수행되고 있다4-6).
미세조직 관점에서 용접금속의 저온인성을 확보하기 위해서는 침상형 페라이트(acicular ferrite)의 분율을 높이는 것이 중요하다. 일반적으로 침상형 페라이트는 결정립 내부에 있는 비금속개재물(non-metallic inclusion)에서 핵생성 되어 사방으로 성장하기 때문에 페라이트 결정립들이 서로 얽혀있는 구조(inter-locking structure)를 갖게 되어 우수한 균열 저항성을 보인다7). 따라서 용접금속에서의 침상형 페라이트 핵생성 기구와 비금속개재물의 역할을 규명하기 위한 연구가 국내외에서 활발하게 진행되고 있다8,9).
침상형 페라이트는 일반적으로 600 MPa 급 용접금속에서 가장 높은 분율로 형성 된다. 합금원소 첨가에 의해 용접금속이 600 MPa 급 이상으로 고강도화되면 속도론(kinetics) 관점에서 침상형 페라이트보다는 베이나이트(bainite), 마르텐사이트(martensite)와 같은 저온변태 조직의 발달이 우세해지기 때문에 용접금속의 저온인성이 급격히 감소하게 된다10,11). 따라서 1 GPa 급 고강도강 용접금속의 저온인성을 확보하기 위해서는 침상형 페라이트의 핵생성에 미치는 비금속개재물의 역할을 명확히 규명하고 이를 바탕으로 한 용접재료의 합금설계가 이루어져야 한다.
본 연구에서는 1 GPa 급 고강도강 용접금속에서의 침상형 페라이트 핵생성 거동을 분석하였다. 용접금속 시편 제작을 위해 인장강도 1 GPa 급의 시험용 플럭스 코어드 와이어(flux cored wire)를 이용하였다. 한편, 일반적으로 후판의 용접부는 열이력에 따라 용착 그대로 (as-depoisted) 상태와 후속 패스에 의해 재가열된 (reheated) 지역으로 구성되어 있으므로 이와 같은 두 지역에서의 침상형 페라이트 형성 거동을 관찰하였다. 먼저 단일 패스 용접을 수행하여 용착 그대로 상태의 용접금속 시편을 제작하였으며, Gleeble simulator를 이용하여 용접금속 재가열부를 재현하였다. 각 시편의 미세조직 및 개재물 분석을 통해 1 GPa 급 용접금속에서의 침상형 페라이트 핵생성기구를 고찰하였다.
2. 실험 방법
용착 그대로 상태의 용접금속 시편을 제작하기 위해 AH36 강판에 5mm 깊이의 V-그루브를 가공한 후 인장강도 1 GPa 급의 시험용 플럭스 코어드 와이어를 이용하여 약 20 kJ/cm의 입열량으로 단일 패스 용접을 실시하였다. 시험용 플럭스 코어드 와이어의 기계적 성질은 패스당 약 20 kJ/cm의 입열량으로 20mm의 두께를 다층 용접 시 인장강도가 1080 MPa, 연신율이 17.4%, -40°C에서의 충격인성이 40.7J을 나타내는 것으로 확인되었다. 광학발광분석기 (optical emission spectroscopy) 및 N/O 분석기를 이용하여 용접금속의 화학조성을 분석한 결과를 Table 1에 제시하였다. 또한 Gleeble simulator를 이용하여 용접금속의 재가열 상태를 재현하여 용착 그대로 상태와 비교하였다. 재가열부 재현을 위한 열사이클의 최고온도(peak tem- perature)는 오스테나이트 영역, 즉 Ac3 이상인 1350°C로 선정하였으며, 20kJ/cm의 용접 입열량을 갖는 조건으로 Rosenthal 방정식을 이용하여 계산하였다12). Fig. 1은 열사이클 계산 결과를 나타낸 것이다.
용착 그대로 및 재가열 상태 시편의 미세조직 분석을 위해 광학현미경 (optical microscopy, OM), 주사전자현미경 (scanning electron microscopy, SEM) 및 전자후방산란회절(electron back-scattered diffraction, EBSD)을 이용하였다. OM 및 SEM 관찰을 위한 시편은 기계적 연마 후 2% nital 용액으로 에칭(etching)하였으며, EBSD 분석을 위한 시편은 5% per- chloric acid + 95% methanol 전해액을 사용하여 전해연마 하였다. 개재물 관찰 및 침상형 페라이트의 핵생성 거동 분석을 위해 투과전자현미경(transmission electron microscopy, TEM), 제한시야회절 (selected area electron diffraction, SAED) 및 에너지분광분석기(energy dispersive spectroscopy, EDS)를 이용하였으며, TEM 분석을 위한 시편은 jet polisher와 집속이온빔(focused ion beam, FIB)을 이용하여 준비하였다.
3. 실험 결과 및 고찰
Fig. 2는 OM을 이용해 관찰한 용착 그대로 및 재가열 상태 시편의 미세조직 관찰 결과를 나타낸 것이다. 두 시편 모두 래스 (lath) 형태의 저온변태 조직으로 구성되었으나, 미세조직 분율 및 분포 양상에 있어서는 큰 차이를 보였다.
미세조직 특성을 명확히 규명하기 위한 고배율의 SEM 관찰 결과를 Fig. 3에 나타내었다. 용착 그대로 상태에서는 베이나이트 기지(matrix)에 고립된 침상형 페라이트가 분산된 형태로 존재하는 것을 확인할 수 있었다. 1개의 개재물로부터 핵생성되어 성장한 페라이트 래스의 수는 1개 혹은 2개이며, 래스의 종횡비(aspect ratio)는 약 10으로 가늘고 긴 형태를 보였다. 이와 같이 1 GPa 급 고강도강 용접금속에서 형성되는 침상형 페라이트의 분포와 형상은 기존에 많은 연구를 통해 보고되고 있는 600 MPa 급 용접금속에서의 그것과는 다소 다르다. 최근 연구9)에서 고찰한 바 있듯이 600 MPa 급 용접금속에서는 일반적으로 3개 이상 다수의 페라이트 래스가 개재물에서 핵생성된 후 사방으로 성장할 뿐만 아니라 1차로 형성된 페라이트 래스의 계면으로부터 또 다른 2차 페라이트가 핵생성되는 공명 핵생성(sympathetic nucleation)도 발생하기 때문에 침상형 페라이트가 결정립 내부 넓은 영역에 걸쳐 고르게 분포하게 된다. 즉 앞서 서론에서 언급한 페라이트 결정립들이 서로 얽혀있는 구조를 갖게 된다. 한편 재가열 상태 시편에서는 침상형 페라이트가 거의 관찰되지 않았으며, coalesced 베이나이트가 발달하였다. 최근 연구13,14)에서 보고한 바 있듯이 coalesced 베이나이트는 방위차가 작은 인접한 래스들이 성장단계에서 유착(coale- scence)됨으로써 형성되기 때문에 래스들이 다른 결정립과 충돌(impingement)없이 성장할 때 형성될 가능성이 높다. 결정립 내부에서 고립된 형태로 형성되는 침상형 페라이트의 경우 래스들의 성장 및 유착을 방해할 것으로 생각되며, 따라서 재가열부에서의 coalesced 베이나이트 조직 발달은 침상형 페라이트 분율 감소의 결과로 해석된다.

SEM micrographs of the weld metals: (a) as-deposited and (b) reheated. AF: acicular ferrite, CB: coalesced bainite. (Solid and dotted circles indicate nucleants and non-nucleants for AF, respectively)
Fig. 4는 두 시편의 EBSD 분석 결과를 나타낸 것으로, 용착 그대로 상태일 때 15°이상의 고경각입계(high- angle grain boundary)로 구분된 유효결정립도(effec- tive grain size)가 작은 것으로 확인되었다. 즉 베이나이트 기지 내에 형성된 침상형 페라이트가 효과적으로 유효결정립 크기를 감소시키는 것으로 판단된다. 여러 연구를 통해 유효결정립도가 작을수록 저온인성이 높아진다는 결과가 확인된 바 있으며1,2,,15), 따라서 1 GPa 급 고강도강 용접금속이 우수한 저온인성을 높이기 위해서는 침상형 페라이트 조직이 필수적일 것으로 판단된다.

IPF map of the weld metals: (a) as-deposited and (b) reheated. (Black line indicates grain boundaries having a misorientation angle of 15° or more)
Fig. 5와 6은 TEM을 이용해 관찰한 용착 그대로 및 재가열 상태 시편의 개재물 관찰 결과를 나타낸 것이다. 일반적으로 용접 후 용접금속 내부에 형성된 액상 비금속개재물은 냉각에 의해 일부는 결정상으로 정출되며 나머지는 과냉각 액상 상태로 존재하다가 비정질상으로 응고하게 된다. 두 시편의 경우도 대부분 개재물은 결정상인 MnTi2O4와 비정질상으로 구성된 복합산화물 형태를 보였으며, 이에 대한 결정구조 등 구체적인 분석결과는 최근연구에서 보고한 바 있다9).
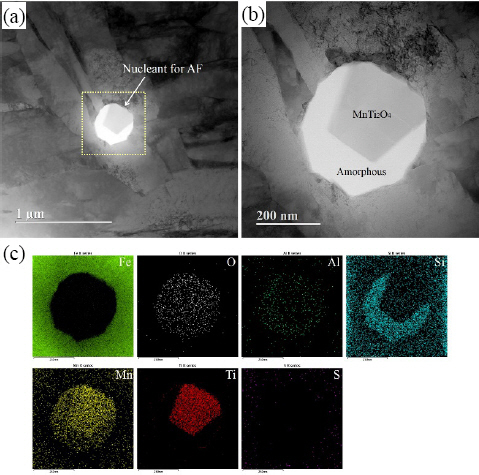
TEM micrographs of different magnification of (a, b) the typical type of inclusions in the as-deposited weld metal and (c) EDS elemental mapping images taken from the dotted area in (a)

TEM micrographs of different magnification of (a, b) the typical type of inclusions in the reheated weld metal and (c) EDS elemental mapping images taken from the dotted area in (a)
Fig. 7에 나타낸 바와 같이 TEM 및 SAED 분석 결과 개재물 표면에 위치한 MnTi2O4와 이로부터 핵생성된 페라이트 래스 사이에 Baker-Nutting 방위관계가 있는 것으로 확인되었다. 이러한 방위관계를 만족할 때 격자 부정합 정도가 약 5.94%로 매우 낮아지는 것으로 확인된 바 있다9). 즉 개재물과 페라이트 래스 사이의 계면에너지 감소로 인해 침상형 페라이트 핵생성을 위한 활성화에너지 장벽이 낮아진 것으로 판단된다. 이러한 ‘격자정합성 이론’은 지금까지 여러 연구에서 제안되어 왔다8,9). TiO, TiN 등 페라이트와 높은 정합성을 가지는 개재물들이 우수한 핵생성 능력을 가진다고 하였다.

Results of TEM analysis of the FIB sample extracted from the as-deposited weld metal: (a) scanning TEM image of the FIB sample (circle indicates the aperture position used to obtain the SAED pattern), (b) SAED pattern of the circular area in (a)
한편 여러 연구자들은 개재물 주변에 형성된 Mn-결핍층(Mn-depleted zone, MDZ)에 의한 열역학적 구동력(driving force)의 증가가 침상형 페라이트 변태를 촉진시킨다고 제안하고 있다6,8,,9). 최근 연구에 따르면 용접금속 기지의 고상선(solidus)보다 낮은 온도에서 개재물 표면에 석출되는 상(phase)의 주변에 MDZ가 형성될 수 있다고 하였다16,17). MnTi2O4와 MnTiO3 등의 석출로 인해 주변 기지의 Mn이 결핍되고 용접금속의 빠른 냉각에 의해 Mn이 균질화(homo-genization)되지 못하면 MDZ가 형성된다는 것이다. Fig. 8과 같이 개재물 표면에 석출된 MnTi2O4로부터 Mn 조성에 대한 EDS 분석을 수행한 결과, 용착 그대로 상태에서는 MDZ가 형성되어 있는 것을 확인할 수 있었다. 따라서 용착 그대로 상태에서 침상형 페라이트가 발달하는 것은 개재물과 페라이트와의 격자 정합성이 높을 뿐만 아니라 개재물 주변에 형성된 MDZ로 인해 개재물의 핵생성 능력이 높아진 결과로 판단된다. 반면 재가열부에서는 MDZ가 관찰되지 않았는데, 이것은 열사이클에 의해 Mn 균질화가 발생했기 때문인 것으로 사료된다17). 앞의 미세조직 관찰 결과에서 확인한 재가열부에서의 침상형 페라이트 분율 감소는 열사이클로 인해 MDZ가 사라짐에 따라 개재물의 핵생성 능력이 감소한 결과로 해석된다.
4. 결 론
저온변태 조직의 발달이 우세한 1 GPa 급 고강도강 용접금속에서 침상형 페라이트를 형성시키기 위해서는 개재물의 핵생성 능력을 극대화시키는 것이 중요하다. 본 연구결과 페라이트와의 격자 정합성이 높은 동시에 주위 기지에 MDZ를 형성하는 개재물이 침상형 페라이트의 핵생성 능력이 매우 높을 것으로 예상되며, 따라서 이러한 개재물을 형성시킬 수 있는 용접재료의 합금성분계를 도출하기 위한 연구가 수행되어야 할 것으로 판단된다. 또한 다층 용접부의 재가열 지역에서 개재물의 핵생성 능력 감소를 방지하기 위한 추가적인 연구도 필요할 것으로 보인다.
후 기
본 연구는 원자력안전위원회의 재원으로 한국원자력안전재단의 지원을 받아 수행한 원자력안전연구사업의 연구결과입니다. (No. 1805005)